What are antibodies?
To understand how and why vaccine reactions occur, it is first
necessary to understand how the immune systemImmune systemA complex system of organs and processes in the body responsible
for fighting disease. Its primary function is to identify foreign
substances in the body (including bacteria, viruses, fungi,
parasites or transplanted organs and tissues) and develop a defense
against them. This defense is known as the immune response. helps to protect the body against infection. It is designed to
identify and destroy harmful foreign organisms (pathogens
PathogenAny disease-causing substance. Most commonly used for organisms
(e.g., bacteria, viruses) and their biological products (e.g.
toxins).) from the body, and neutralize the toxins (poisons) that some bacteria
produce.

Source: wikipedia.org
The pathogens causing the vaccine-preventable diseasesVaccine-preventable diseasesDiseases for which vaccines exist that can confer partial or
complete protection. described in this module are mainly microorganisms
MicroorganismsTiny organisms (including bacteria and viruses) that can only be
seen with a microscope. such as bacteria
BacteriaSingle-celled life-forms that can reproduce quickly on their own.
Some bacteria cause disease. or viruses
VirusAn ultramicroscopic infectious agent that consists of genetic
material surrounded by a protein coat. A virus can replicate
themselves only within cells of living hosts.
- Bacteria are single-celled life-forms that can reproduce quickly on their own.
- Viruses, on the other hand, cannot reproduce on their own. They are ultramicroscopic infectious agents that replicate themselves only within cells of living hosts.

The immune system responds to bacteria and viruses in a very complex
way: it recognizes unique molecules (antigensAntigenA foreign substance in the body that triggers the production of
antibodies.) from bacteria and viruses and produces antibodies (a type of protein)
and special white blood cells called lymphocytes that mark the antigens
for destruction.
During the primary immune responseImmune responseThe body's defense against foreign objects or organisms, such as
bacteria, viruses or transplanted organs or tissue. to the first encounter with a specific pathogen, some lymphocytes
called memory cells develop with the ability to confer
long-lasting immunity
ImmunityThe body's response mechanism for fighting against bacteria,
viruses and other foreign substances. If a cell or tissue (such as
bacteria or a transplanted organ) is recognized as not belonging to
the body, the immune system will act against the "invader." The
immune system is the body's way to fight external invasions. to that pathogen, often for life. These memory cells recognize
antigens on the pathogens they have encountered before, triggering the
immune system to respond faster and more effectively than on the first
exposure.

Source 1: wikipedia.org, Source 2: wikipedia.org
The graph below compares the primary and secondary immune responses to the same pathogen. The secondary response may eliminate the pathogens before any damage occurs.59

The goal of all vaccines is to elicit an immune response against an
antigen so that when the individual is again exposed to the antigen,
a much stronger secondary immune responseImmune responseThe body's defense against foreign objects or organisms, such
as bacteria, viruses or transplanted organs or tissue. will result. Vaccines contain the same antigens that are
found on pathogens that cause the associated disease, but exposure
to the antigens in vaccines is controlled. By priming
PrimingThe process of artificial induction of immunity, in order to
protect against infectious disease. Priming the immune system
involves sensitizing or stimulating an immune response with an
antigen that can produce immunity to a disease-causing organism
or toxin (poison). Vaccinations involve the administration of
one or more of these antigens, which can be administered in
several forms. the immune system through vaccination, when the vaccinated
individual is later exposed to the live pathogens in the
environment, the immune system can destroy them before they can
cause disease.
Thus, there are two ways of acquiring immunity to a pathogen — by
natural infection and by vaccination. Natural infections and
vaccines produce a very similar end result — immunity — but the
person who receives a vaccine does not endure the illness and its
potential life-threatening complications. The very low risk of an
adverse event caused by a vaccine greatly outweighs the riskRiskThe probability that an individual will experience a certain
event during a defined period of time. of illness and complications caused by natural infection. The
following pages will discuss in further detail the attributes of
vaccines and the characteristic causes for adverse events.

Vaccines to prevent other diseases have become available since the introduction of EPI and are recommended by the WHO for global use. They cover diseases such as hepatitis B disease, infections or cervical cancer caused by human papillomavirus, diarrhoeal disease caused by rotaviruses, and pneumonia and other respiratory tract infections caused by Haemophilus influenzae type B and pneumococcal bacteria. Others, such as the vaccine against yellow fever, are recommended in countries where the disease burden is significant.
The main vaccine-preventable diseases and the associated vaccines
Tubercle bacillus | Bacillus Calmette-Guérin (BCG) vaccine |
Poliovirus |
Oral polio vaccine (OPV) vaccine, Inactivated polio vaccine (IPV) vaccine |
Corynebacterium diphtheriae (Diphtheria)* |
Diphtheria toxoid** vaccine |
Clostridium tetani (Tetanus)* |
Tetanus toxoid (TT) vaccine |
Pertussis* |
Whole-cell pertussis (wP) vaccine, Acellular (cell-free) pertussis (aP) vaccine |
Measles virus | Measles vaccine |
Hepatitis B virus | Hepatitis B vaccine |
Rotavirus | Rotavirus vaccine |
Haemophilus influenzae type B (Hib) | Hib conjugate vaccine |
Streptococcus Pneumoniae (Pneumococcal infection) |
Pneumococcal vaccines |
Yellow fever virus | Yellow fever vaccine |
Human Papillomavirus | Human Papillomavirus (HPV) vaccine |
Hepatitis A virus | Hepatitis A vaccine |
Varicella-Zoster virus | Varicella Zoster virus (VZV) vaccine |
* DiphtheriaDiphtheria toxoid vaccineA vaccine containing diphtheria toxoid, used to immunize
against diphtheria., tetanus
Tetanus toxoid (TT) vaccineA preparation of tetanus toxoid used to immunize against
tetanus. When given to women of childbearing age, vaccines
that contain tetanus toxoid (TT or Td) not only protect
women against tetanus, but also prevent neonatal tetanus
in their newborn infants. and pertussis
Pertussis vaccineTwo types of pertussis vaccines are currently available:
the inactivated whole-cell vaccine (wP) and subunit
protein-based vaccine (aP). vaccines are usually administered in combination
vaccines (e.g. DTwP, DTaP) when given to infants and young
children. These vaccines are also available in combinations
with hepatitis B
Hepatitis B vaccine (HepB)A subunit protein-based recombinant vaccine used against
hepatitis B infection. (e.g. DTwP-HepB, DTaP-HepB) and/or Hib
Haemophilus influenzae type b (Hib) vaccineA subunit polysaccaride-conjugate vaccine used to
immunize against invasive Hib disease. vaccines (e.g. DTPwP-HepB+Hib, DTPaP-HepB+Hib).
**Diphtheria toxoidDiphtheria toxoid vaccineA vaccine containing diphtheria toxoid, used to immunize
against diphtheria. is only available as a combination.
TYPES OF VACCINE
There are many types of vaccines, categorized by the antigen used in their preparation. Their formulations affect how they are used, how they are stored, and how they are administered. The globally recommended vaccines discussed in this module fall into the four main antigen types shown in the diagram.

Vaccine manufacturers strive to develop vaccines that:
- are effective in preventing or reducing severity of infectious disease;
- provide durable, long-term protection against the disease;
- achieve immunity with a minimal number of doses;
- provide the maximum number of antigens that confer the broadest protection against infection;
- cause no or mild adverse events;
- are stable at extremes of storage conditions over a prolonged period of time;
- are available for general use through mass production;
- are affordable to populations at risk for infectious disease.
The three functions of antibodies
Antibodies have three main functions:
1) Antibodies are secreted into the blood and mucosa, where they bind
to and inactivate foreign substances such as pathogens and toxins
(neutralization).
2) Antibodies activate the complement system to
destroy bacterial cells by lysis (punching holes in the cell wall).
3)
Antibodies facilitate phagocytosis of foreign substances by phagocytic
cells (opsonization).
Antibodies and the four key features of the immune system
1. Specificity of antibodies: Antibodies
precisely recognize toxins and pathogens.
2. Diversity of antibodies: Antibodies against a variety of antigens preexist in the body.
3. Immunological memory: We don’t don’t develop
symptoms of measles
4. Immune tolerance: Self cells and tissues are
not normally attacked.
The specificity of antibodies
Each antibody recognizes one specific antigen.
For example, an
antibody that recognizes the mumps virus cannot recognize the measles
virus. Conversely, an antibody that recognizes the measles virus
cannot recognize the mumps virus. This feature is called “antibody
specificity.”
Each B cell (antibody-producing cell)
produces one kind of antibody. However, pathogens produce millions of
harmful factors.
Then, how does the body defend itself
against countless harmful factors?
The diversity of antibodies and immunological memory
Tens to hundreds of millions of different B cells are circulating in
the body so that every antigen is recognized. In other words, the body
is prepared for the invasion of pathogens by possessing B cells that
produce unique antibody molecules. This feature is called “antibody
diversity.”
After an infection, the cells producing
pathogen-specific antibodies multiply and increase proportionally. As
a result, the body is protected from repeated infection. This feature
is called “immunological memory.”
Gene rearrangement
Antibody-producing B cells are produced in the bone marrow and mature
in the periphery. During B-cell maturation, the antibody genes
(immunoglobulin genes) undergo recombination, generating an enormous
repertoire of antigen-binding sites (the variable region). This
phenomenon is called “gene rearrangement.”
The gene locus encoding the H chain variable region:
The locus
contains an array of about 100-300 V gene segments,
about 25 D gene segments, and
6 J gene segments. One each of
the V, D, and J gene
segments are selected and joined together.
The gene locus encoding the L chain variable region:
There are
two loci: κ and λ.
The Îş locus consists of an array of about
40 V and 5 J gene segments. The
λ locus consists of an array of about 30 V and
4 J gene segments. One each of
the V and J gene segments are
selected and joined together.
Immunoglobulins (antibodies)
to countless antigens are produced from a limited number of genes by
recombination of gene segments. Gene rearrangements also occur during
T cell maturation in the thymus.
Immune tolerance
“Why don’t tens to hundreds of millions of B cells recognize and
attack self-tissues?”
Antibodies recognize all types of
antigens, except self-antigens. This feature is called “immune
tolerance.” B cells that react to self-antigens are generated, but are
eliminated within the bone marrow. Even if some autoreactive B cells
evade the elimination process and reach the periphery, those B cells
that produce antibodies to self-antigens (autoantibodies) are
inactivated by another mechanism including regulation by Tregs..
When
these mechanisms are disrupted, “autoimmune disease” develops,
characterized by immune cell-mediated self-tissue attack. Possible
causes of autoimmune disease include viral infection, high fever,
pregnancy, and the recently proposed abnormalities in the intestinal
microbiome. However, the details of the mechanism remain unknown.
List of autoimmune diseases
Structure and characteristics of antibody isotypes
Human antibodies are classified into five isotypes (IgM, IgD, IgG, IgA, and IgE) according to their H chains, which provide each isotype with distinct characteristics and roles.
IgG
IgG is the most abundant antibody isotype
in the blood (plasma), accounting for 70-75% of human immunoglobulins
(antibodies). IgG detoxifies harmful substances and is important in
the recognition of antigen-antibody complexes by leukocytes and
macrophages. IgG is transferred to the fetus through the placenta and
protects the infant until its own immune system is functional.
IgM
IgM usually circulates in the blood,
accounting for about 10% of human immunoglobulins. IgM has a
pentameric structure in which five basic Y-shaped molecules are linked
together. B cells produce IgM first in response to microbial
infection/antigen invasion.
Although IgM has a lower affinity for
antigens than IgG, it has higher avidity for antigens because of its
pentameric/hexameric structure. IgM, by binding to the cell surface
receptor, also activates cell signaling pathways.
IgA
IgA is abundant in serum, nasal mucus,
saliva, breast milk, and intestinal fluid, accounting for 10-15% of
human immunoglobulins. IgA forms dimers (i.e., two IgA monomers joined
together). IgA in breast milk protects the gastrointestinal tract of
neonates from pathogens.
IgE
IgE is present in minute amounts,
accounting for no more than 0.001% of human immunoglobulins. Its
original role is to protect against parasites. In regions where
parasitic infection is rare, IgE is primarily involved in
allergy.
IgD
IgD accounts for less than 1% of human
immunoglobulins. IgD may be involved in the induction of antibody
production in B cells, but its exact function remains unknown.
Immunoglobulin class switching
B cells expressing plasma membrane-bound IgM and IgD (mature B cells) are activated upon encounter with a specific antigen, and begin to proliferate and produce secretory IgM and IgD. With further activation by the antigen or other stimuli, these mature B cells differentiate into cells that produce increasing amounts of secreted immunoglobulins, and start to produce immunoglobulin isotypes other than IgM and IgD. This process is called “immunoglobulin class switching”.
Factors in the B cell environment (including hormones secreted by T cells [cytokines]) direct the isotype switching.
Examples
Interleukin 4 (IL4) stimulates class switching from IgM/IgD to IgG1
and IgE
Interleukin 5 (IL5) stimulates class switching from
IgM/IgD to IgA
*Interleukins are a type of cytokine that is
produced by leukocytes and lymphocytes during the immune
response.
How to generate polyclonal antibodies
Overview of the procedure
An antigen (immunogen) injected into animals
induces them to produce and secrete high levels of antibodies into the
blood.
Several months after repeated immunization, the blood
(plasma, serum) is collected, and antibodies are purified (by methods
described later). The antibodies generated by this method are called
polyclonal antibodies because they are derived from different B cell
clones and the resulting antiserum contains numerous different
antibodies that react to the injected immunogen.
Animals used for immunization (immunized animals)
In addition to mice and rabbits, various mammals and birds are used for
immunization, including rats, hamsters, guinea pigs, chickens, goats,
sheep, and donkeys.
Immunoglobulin isotypes, organization of immunoglobulin genes, mechanism of diversification, and the organ sites of antibody diversification differ between vertebrate animals.
How antibodies are produced
After immunization, blood IgM levels increase first.
With repeated
immunization, IgG levels increase.
The affinity (binding ability) of IgM for the antigen does not increase
even after repeated immunization.
In contrast, the
binding ability of IgG increases with repeated immunization.

Extending the immunization period, in the hopes of developing a higher affinity antibody to the antigen, is not necessarily beneficial. After an excessively long immunization period, non-specific antibodies, including self-reactive antibodies, increase in amount and the animals’ health may deteriorate. To generate high quality antibodies, it is important to periodically collect a small amount of blood and evaluate the affinity of the antibody for the antigen and non-specific reactions.
How to produce monoclonal antibodies
Overview of the procedure
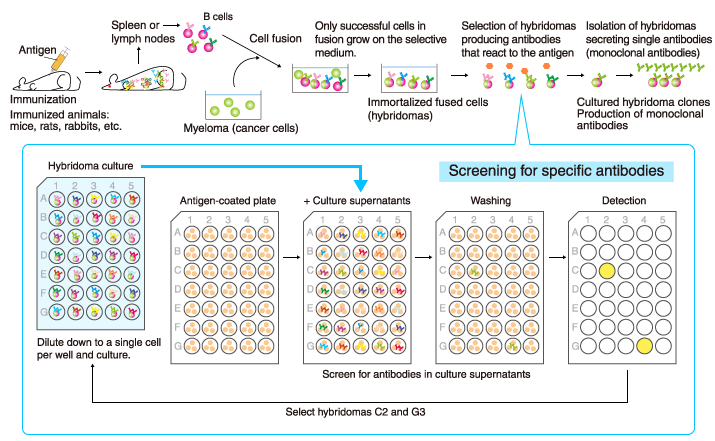

A single antibody (monoclonal antibody) can be stably produced if a
single B cell producing the antibody is isolated and cultured
indefinitely.
This is achieved by artificially fusing the
antibody-producing B cells with immortalized cancer cells (myeloma) to
generate hybridomas that live indefinitely and contain genes encoding
specific antibodies, and by selecting the hybridoma clones that produce
the desired monoclonal antibodies with high affinity and specificity.
It
usually takes 4-6 months from immunization of animals to production of
monoclonal antibodies.
Difference between clones in monoclonal antibodies
Even though monoclonal antibodies against the same antigen are produced
each clone reacts to different epitopes on the same antigen Also, each
clone have different suitable applications. It is important to take note
of the antigen name and clone name when selecting a monoclonal
antibody.
Phage display method
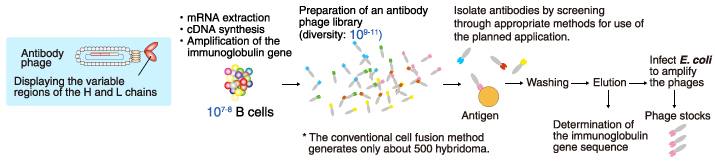

A phage (bacteriophage) is a virus that infects bacteria. The phage
genetically integrated with genes coding a part of antibody is referred
to an antibody phage, and displays on their surface the antibody
fragment, such as Fab form or scFv form. An antibody phage library is a
huge mixed population of antibody phages that can react with various
molecules. In the phage display method, antibodies against the target
molecule are selected from the antibody phage library by using a
molecular affinity of antibodies and the target molecule.
Various sources of the immunoglobulin gene are used in the antibody
phage library. For example, naĂŻve antibody phage libraries are prepared
using the B cells of healthy individuals. Other examples include B cells
from patients whose sera contain antibodies that potently neutralize
pathogens or toxins, and B cells from animals immunized with a specific
antigen.
Difference between polyclonal and monoclonal antibodies
Polyclonal antibodies | Monoclonal antibodies | |
---|---|---|
Animal species |
Rabbit, guinea pig, goat, sheep, rat, mouse, chicken, etc. | Rat, mouse, chicken, rabbit, human, etc. |
Form |
Antiserum | Hybridoma |
Class, subclass | Mixed classes | Single class |
Epitope | React to multiple epitopes | React to a single epitope |
Specificity |
Lower than monoclonal antibodies because multiple types of
antibodies are present. |
High if good quality antibodies are selected. |
Reproducibility | Variable among lots. | The same antibodies are produced indefinitely. |
Stability |
Binding ability tends to be unaffected by fixation/denaturation
of the antigen, because multiple different antibody molecules
are present. Tolerate modifications, such as labeling and removal of the Fc region. |
Binding ability may be lost if the epitope is lost by
fixation/denaturation of the antigen, because monoclonal
antibodies are homogeneous. Tend to be sensitive to modifications, such as labeling and removal of the Fc region. |
Antigen binding
Antigen bindingMonoclonal antibody reacts to a single epitope.
Therefore, only one antibody molecule can bind to an antigen
molecule.
In contrast, polyclonal antibody is a collection of
immunoglobulin molecules that react against a specific antigen, each
recognizes a different epitope. Therefore, multiple antibody molecules
bind to an antigen molecule. (if sufficiently large).
Labeled (secondary) antibodies
Similarly, only one antibody molecule can bind to a primary antibody
molecule if the secondary antibody is a labeled monoclonal antibody.
Whereas multiple antibodies can bind to a primary antibody molecule if
the secondary antibody is a polyclonal antibody.
Consequently, polyclonal antibodies provide a higher sensitivity of
detection (amplification of the signal) and, therefore, are commonly
used as a secondary antibody.
Sensitivity to protein-degrading enzymes (proteases)
Proteases are often used for antibody modification in order to add a
label, or to remove the Fc region to reduce non-specific reaction.
The
high sensitivity of monoclonal antibodies to protease digestion can
result in digestion outside the intended regions, or loss of
antigen-binding ability. Conversely, if the antibody is resistant to the
protease, intended modifications would be difficult.
In
contrast, sensitivity to proteases is unlikely to cause a problem with
polyclonal antibodies, and antigen-binding ability tends to be
unaffected because multiple different antibody molecules are present.
Thus, polyclonal antibodies are amenable to modification.
How to purify antibodies
Antibodies are usually purified by the following three steps.
1) Partially remove solid materials and proteins other than the
antibodies.
Perform centrifugation or filtration.
2) Isolate antibodies by affinity chromatography (purification with Protein A/G / antigen-affinity purification).
3) Remove contaminants remaining after step 2).
Perform gel
filtration chromatography to further purify the antibodies and exchange
the buffer.
Purification with protein A/G
IgG can be purified using a column packed with immobilized Protein A or Protein G.
Protein A is a cell wall protein of Staphylococcus aureusthat specifically binds to the Fc region of mammalian IgG. Staphylococcus aureus is a commensal bacterium of the skin and the gastrointestinal
tract of humans and other animals, and occasionally causes an infectious
disease and food poisoning. Protein A captures IgG, allowing the
bacteria to evade elimination by the immune system.
*Protein G: A cell wall protein isolated from group G Streptococci
The ability of Protein G and Protein A to bind to Ig differs between Ig subclasses and between species. The use of Protein G or A should depend on the purpose.
IgM and IgY are purified by different columns.
Antigen-affinity purification
Affinity chromatography is performed using a column packed with
immobilized antigen, the same antigen used for immunization. Only
antibodies that bind to the antigen are isolated.
This method provides a higher yield of antigen-specific antibodies than
Protein A/G affinity chromatography, though the total amount of
recovered antibodies is lower.
Protein A/G affinity chromatography and antigen-affinity chromatography cannot be used if the antibodies are inactivated in an acidic solution. In that case, antibodies are purified by ammonium sulfate precipitation (salting out) or ion exchange chromatography.
https://www.mblbio.com/bio/g/support/method/index.html
>> Details of antibody isotypes and subclasses
>> Details of affinity chromatography
>> Details of gel filtration chromatography
Humoral Immune Response
Clonal Selection and B-Cell Differentiation
B cells mature in the bone marrow, where they undergo VDJ recombination to produce unique receptors that do not react to self-antigens.
Learning Objectives
Describe clonal selection and B cell differentiation
Key Takeaways
Key Points
- In the bone marrow, central tolerance of B cells is produced through negative selection. Immature B cells are tested for auto-reactivity before leaving the bone marrow. The immature B cells whose receptors (BCRs) bind too strongly to self antigens will not be killed.
- When the B cell receptor on the cell surface matches its cognate antigen in the body, the B cell proliferates and secretes a free form of those receptors ( antibodies ), with identical binding sites as on the original cell surface.
- B cells that have not been exposed to antigen, also known as naĂŻve B cells, can be activated in a T cell-dependent or independent manner.
- There are two types of T cell independent activation: Type 1 T cell-independent (polyclonal) activation, and type 2 T cell-independent activation.
- During clonal selection, random mutations during clonal expansion cause the production of B cells with increased antibody-binding affinity for their antigens.
- The clonal selection hypothesis may explain why secondary immune responses are so effective at preventing reinfection by the same pathogen.
Key Terms
- Clonal selection: The theory that lymphocytes bear antigen receptors before activation and that random mutations during clonal expansion cause the development of lymphocytes with high binding affinities for their antigens.
- humoral: Of or relating to the body fluids or humors.
- memory B cell: A B cell subtype formed following primary infection in which the cell recognizes a specific epitope.
B cells are lymphocytes that play a large role in the humoral immune response (as opposed to the cell-mediated immune response, which is governed by T cells). B cells primarily function to make antibodies against antigens, act as antigen-presenting cells (APCs), and eventually develop into memory B cells to provide long-term immunity. B cells undergo clonal selection and develop similarly to T cells with some notable differences.
B Cell Development
Immature B cells are produced in the bone marrow of most mammals. Their development occurs through several stages, each representing a change in the genome content at the antibody loci. An antibody is composed of two identical light (L) and two identical heavy (H) chains, and the genes specifying them are found in the V (variable) region and C (constant) region. The heavy-chain V region has three segments, V, D and J. These segments recombine randomly in a process called VDJ recombination to produce a unique variable domain in the immunoglobulin of each individual B cell.
Similar rearrangements occur for the light-chain V region but with only two segments involved: V and J. When the B cell fails in any step of the maturation process, it will die by apoptosis, here called clonal deletion. This is a form of positive selection. B cells are also tested for autoreactivity through negative selection. If these B cells have high affinity for binding to self-antigens, they will die by clonal deletion or another pathway such as anergy.
B Cell Activation
B cell activation refers to the differentiation and clonal expansion of B cells.When the B cell receptor on the cell surface matches its cognate antigen in the body, the B cell proliferates and secretes a free form of those receptors (antibodies) in the body, with binding sites identical to those on the original cell surface. However, B cell recognition of antigens is not the only element necessary for B cell activation. B cells that have not been exposed to antigen, also known as naĂŻve B cells, can be activated in a T cell-dependent or independent manner.
- T cell dependent activation is activation of B cells by type 2 helper T cells in the lymph nodes.
- T cell independent activation occurs when antigens directly bind to B cell themselves, usually through cross-linking the antigen to the B cell receptor or receiving the antigen with a toll-like receptor.
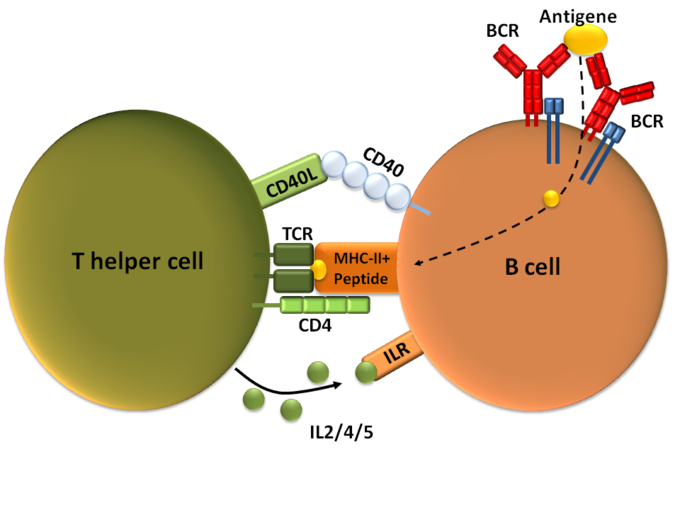
T-dependent B cell activation: T cell-dependent B cell activation, showing a TH2-cell (left), B cell (right), and several interaction molecules.
B Cell Differentiation and Clonal Expansion
After activation, the B cell undergoes differentiation and clonal expansion, which usually involves migration to germinal centers if the activation takes place in a lymph node. B cell differentiation is the process by which B cells change into different types, such as plasma cells and plasma blasts. Clonal expansion is the process by which daughter cells arise from a parent cell. During B cell clonal expansion, many copies of that B cell are produced that share affinity with and specificity of the same antigen.
Clonal Selection
Clonal selection is a theory stating that B cells express antigen-specific receptors before antigens are ever encountered in the body. After B cell activation, the B cells clone themselves through clonal expansion, but during each cellular division, random mutations occur that gradually increase the binding affinity for B cell-produced antibodies to antigens.
For example, memory B cells that differentiate after an adaptive immune response are thought to undergo clonal selection so that antibodies produced by newer memory B cells have considerably higher binding affinities to their antigens. This theory may explain why secondary immune responses from memory cells are so effective that repeated infections by the same pathogen are stopped before symptoms even develop. Following the initial infection, random mutations during clonal selection could produce memory B cells that can more easily bind to antigens than can the original B cells.
Structure and Function of Antibodies
An antibody is a Y-shaped protein produced by B cells to identify and neutralize antigens in the body.
Learning Objectives
Describe antibody structure and function
Key Takeaways
Key Points
- An antibody, also known as an immunoglobulin, is a large Y-shaped protein produced by B- cells and used by the immune system to identify and neutralize foreign objects such as bacteria and viruses.
- Each tip of the “Y” of an antibody contains a paratope (a structure analogous to a lock) that is specific for one particular epitope (similarly analogous to a key) on an antigen, allowing these two structures to bind together with precision.
- Though the general structure of antibodies is very similar, a small region at the tip of the protein is extremely variable, allowing millions of antibodies with different antigen binding sites to exist. This region is known as the hypervariable region.
- Five isotypes of antibodies are found in different locations and perform different specific functions.
- The base of the Y plays a role in modulating immune cell activity. This region is called the Fc region, and phagocytes may bind to it to initiate phagocytosis.
- Antibodies that bind to surface antigens on a bacterium attract the first component of the complement cascade with their Fc region and initiate activation of the classical complement system.
Key Terms
- epitope: Part of a biomolecule (such as a protein) that is the target of an immune response.
- paratope: Part of the molecule of an antibody that binds to an antigen.
- isotype: A marker corresponding to an antigen found in all members of a subclass of a specific class of immunoglobulins.
An antibody (formally called immunoglobulin) is a large Y-shaped glycoprotein produced by B-cells and used by the immune system to identify and neutralize pathogens. Antibodies are produced by B cells, and are either secreted into circulation or remain expressed on the surface of the B cell.
Structure of Antibodies
The antibody recognizes a unique part of an antigen (foreign object). Each tip of the “Y” of an antibody contains a paratope (a structure analogous to a lock) that is specific for one particular epitope (similarly analogous to a key) on an antigen, allowing these two structures to bind together with precision. Using this binding mechanism, an antibody can neutralize its target directly or tag it for attack by other parts of the immune system.
Antibody: Each antibody binds to a specific antigen, an interaction similar to a lock and key.
Antibodies are glycoproteins belonging to the immunoglobulin superfamily, typically made of basic structural units each with two large heavy chains and two small light chains. Most antibodies exist as a monomer, in which they have a single “Y” shaped sub-unit, but some antibodies can exist as dimers (two subunits) or pentamers (five subunits). The paratope is considered a hypervariable region and has the same specificity and antigen-binding affinity as the B cell receptor of the B cell that created the antibody. In some isotypes, the tail end of the antibody is called the constant region and faces away from the “Y-shaped” paratobe ends, functioning as an Fc tail to which phagocytes can bind.
Isotypes
Five different isotypes of antibodies each perform different functions and are generally found in different parts of the body.
- IgA: A dimer secreted into mucosal surfaces, such as the gut, respiratory tract, and urogenital tract, that prevents mucosal invasion into the body by pathogens. It is resistant to the proteolytic enzymes found in the gastrointestinal mucosae.
- IgD: Functions mainly as an antigen receptor on B cells that have not been exposed to antigens. It has been shown to activate basophils and mast cells to produce antimicrobial factors.
- IgE: Found in circulation and binds to allergens, triggering histamine release from mast cells and basophils. Also protects against parasitic worms.
- IgG: Has four different forms and provides the majority of antibody-based immunity against invading pathogens as the best opsonin of any type of antibody. This is because it expresses a tail for Fc receptors on phagocytes to bind to, which activates phagocytosis. It is the only antibody capable of crossing the placenta to give passive immunity to fetus, and can activate the classical complement system.
- IgM: Expressed on the surface of B cells (monomer) and in a secreted pentamer with very high avidity. Eliminates pathogens in the early stages of B cell-mediated (humoral) immunity before there is sufficient IgG. Like IgG, it can also activate the classical complement system.
Function of Antibodies
Circulating antibodies are produced by clonal B cells that specifically respond to only one antigen. Antibodies contribute to immunity in three ways: preventing pathogens from entering or damaging cells by binding to them (neutralization); stimulating removal of pathogens by macrophages and other cells by coating the pathogen (opsonization); and triggering destruction of pathogens by stimulating other immune responses such as the complement pathway. The complement system starts a long cascade of protein productions that either opsonize a pathogen for phagocytosis or lyse it directly by forming a membrane attack complex. During opsonization, the antibody expresses the tail for an Fc receptor on a macrophage, neutrophil, or natural killer cell. The immune cell will then bind to the antibody’s Fc tail instead of the pathogen itself, which speeds up the process of finding pathogens to phagocytize. Additionally, because antibodies have two or more paratopes, they can sometimes link pathogens together, making phagocytosis more efficient.
Role of the Complement System in Immunity
The complement system is the ability of antibodies and phagocytic cells to remove pathogens from an organism.
Learning Objectives
Describe the role of the complement system in immunity
Key Takeaways
Key Points
- The complement system helps antibodies and phagocytic cells clear pathogens from an organism.
- The complement system consists of a number of small proteins produced by the acute phase reaction in the liver during inflammation.
- The complement system might play a role in diseases with an immune component and those of the central nervous system. Complement protein deficiency is a form of primary immunodeficiency.
- The classical complement pathway starts with antibody binding, which causes a cascade reaction of complement proteins that gradually form a membrane attack complex.
- The alternative complement pathway is usually stimulated by pathogen antigens or toxins rather than antibodies, and cleaves C3 until there is enough to continue the steps of the classical complement pathway from the C5 convertase step.
- The lectin pathway is homologous to the classical pathway, but with the opsonin, mannose-binding lectin (MBL), and ficolins, instead of C1 from the antibody. This pathway uses proteases on the MBL to form C3 convertase, which continues the steps of the classical complement pathway from the C3 convertase step.
- The complement system is regulated by complement control proteins, such as decay accelerating pathway, which prevent complement proteins from forming MAC on the body’s cells.
Key Terms
- C5a: A complement protein that is an acute phase inflammatory mediator, causing vasodilation and neutrophil chemotaxis.
- membrane attack complex: The final complex of all complement system pathways that lyses the pathogen. It is composed of C5b, C6, C7, C8, and C9.
- mannan-binding-lectin: A protein that binds to carbohydrates on pathogens to activate the lectin complement pathway.
The complement system or “complements” the ability of antibodies and phagocytic cells to clear pathogens from an organism. The complement system consists of a number of small proteins found in the blood, generally synthesized by the liver as a part of the acute phase reaction during systemic inflammation (from TNF-alpha release). When stimulated by one of several triggers, proteases in the system cleave specific proteins to release cytokines and initiate an amplifying cascade of further cleavages. The end result of this activation cascade is massive amplification of the response and activation of the cell-killing membrane attack complex (also called MAC). There are three different pathways by which the complement system may occur.
Classical Complement Pathway
The classical complement pathway is the main pathway by which the complement system occurs. It is comprised of a cascade of many steps with complement proteins cleaving one another in a sequential order:
- The antibody binds to an antigen on the surface of a pathogen, activating the C1 complement protein.
- C1 acts a protease and cleaves C2 and C4 to form C4b2b.
- C42b converts C3 into C3a and C3b, which forms a C5 convertase.
- C5 convertase cleaves C5 into C5a and C5b.
- C5b forms a complex with C6, C7, and then C8, and C9, which becomes the membrane attack complex that lyses the pathogen.
Note that C5a has a number of other functions in the immune system, such as causing vasodilation during inflammation and stimulating neutrophil chemotaxis. Additionally, the body’s cells express a glycoprotein called decay accelerating factor, which decays C3 and C5 convertase on the body’s cells. This factor prevents membrane attack complexes from forming on the body’s cells under normal conditions.
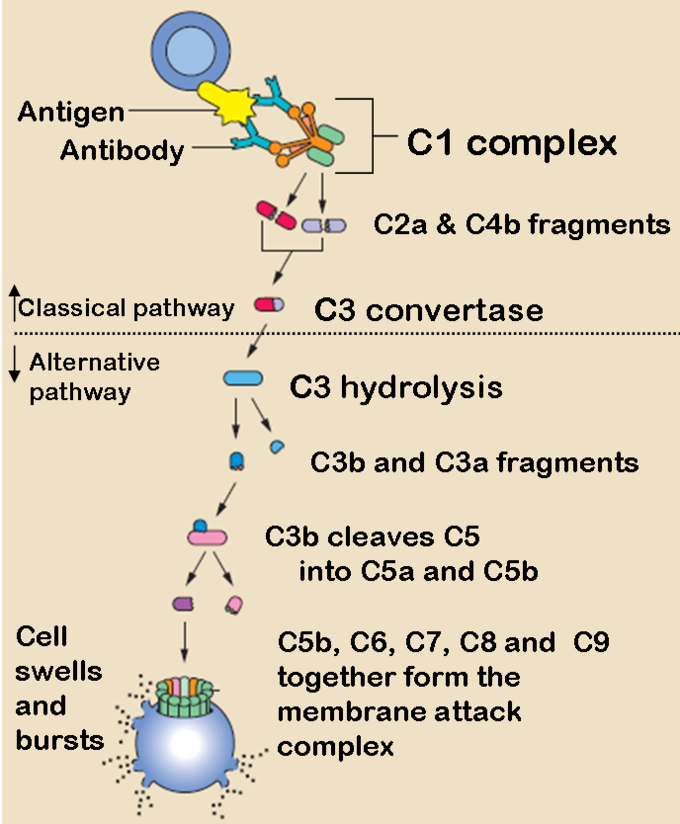
The Classical and Alternative Complement Pathways: The classical and alternative complement pathways start off differently, but end in the same cascade of complement proteins that combine to form a membrane attack complex.
The Alternative Complement Pathway
The alternative pathway may be a leftover evolutionary precursor to the classical pathway. Unlike the classical pathway, the alternative pathway is generally activated by microbial inflammatory mediators instead of antibodies. For example, lipopolysaccharide, the toxin of gram-negative bacteria, may activate this pathway. The steps for the alternative pathway are:
- The pathogenic antigen (such as LPS) activates C3 so it creates a C3B complex
- Factor D cleaves the C3B complex so that C3bBb is created.
- C3bBb is a C3 convertase, which converts more C3 into C3a and C3b.
- Similarly to the classical pathway, C3b forms a C42b complex, and the rest of the steps are essentially the same as the classical pathway, ending with C5b forming a membrane attack complex with C6, C7, C8, and C9.
Lectin Pathway
The lectin pathway is not caused by antibody binding, but by a carbohydrate -binding-protein called mannan-binding-lectin (MBL). It is an acute phase reactant produced in the liver and binds to the carbohydrates on the surfaces of many pathogens. The steps for the lectin pathway are:
- MBL binds to the carbohydrates on a pathogen.
- Proteases bound on the other side of the MBL cleaves C4 into C4a and C4b.
- C4b creates C3 convertase, and the rest of the steps happen identically to the classical pathway from the C3 convertase step.
Problems with the Complement System
The complement system might play a role in diseases with an immune component, such as Barraquer-Simons Syndrome, asthma, lupus erythematosus, glomerulonephritis, various forms of arthritis, autoimmune heart disease, multiple sclerosis, inflammatory bowel disease, ischemia-reperfusion injuries, and rejection of transplanted organs. The complement system is also becoming increasingly implicated in diseases of the central nervous system such as Alzheimer’s disease and other neurodegenerative conditions such as spinal cord injuries. Additionally, deficiencies in complement proteins produced in the liver can lead to a form of primary (congenital) immunodeficiency, in which the body is more susceptible to disease, particularly autoimmune diseases and severe bacterial infections.
Immunological Memory
Immunological memory refers to the ability of B and T cells to produce long-lived memory cells that defend against specific pathogens.
Learning Objectives
Describe immunological memory of the immune system
Key Takeaways
Key Points
- When B and T cells begin to replicate, some offspring will become long-lived memory cells.
- Memory cells remember all specific pathogens encountered during the animal’s lifetime and can thus call forth a strong response if the pathogen ever invades the body again.
- Passive immunity comes from IgG antibodies given through the mother during fetal development and through breast milk. This memory is short term, but protects the infant until its own adaptive immune system is functional.
- During a secondary immune response, memory B and T cells work to rapidly eliminate the pathogen, preventing reinfection by the same pathogen.
- During a vaccination, the antigen of a pathogen is introduced into the body through a weakened form of the pathogen that cannot cause an infection. This stimulates the immune system to develop a specific immunity against that pathogen without actually causing the disease that the pathogen brings.
- Vaccines do not exist for every pathogen due to frequent strain mutations and challenges in producing an immunization strong enough to work, but not strong enough to cause an infection.
Key Terms
- secondary immune response: The act of exposure to the same pathogen after the initial immune response. Memory B and T cells work to rapidly eliminate the pathogen to prevent reinfection.
- vaccination: Inoculation with the weakened form of a pathogen to protect against a particular disease or strain of disease by stimulating the development of immunological memory against that pathogen.
When B and T cells begin to replicate during an adaptive immune response, some offspring become long-lived memory cells. These memory cells remember all specific pathogens encountered during the animal’s lifetime and can thus call forth a stronger response, called the secondary immune response, if the pathogen ever invades the body again. The adaptive immune system is so-named because it is a result of an adaptation to an infection. Immunological memory can either exist in active long-term memory or passive short-term memory.
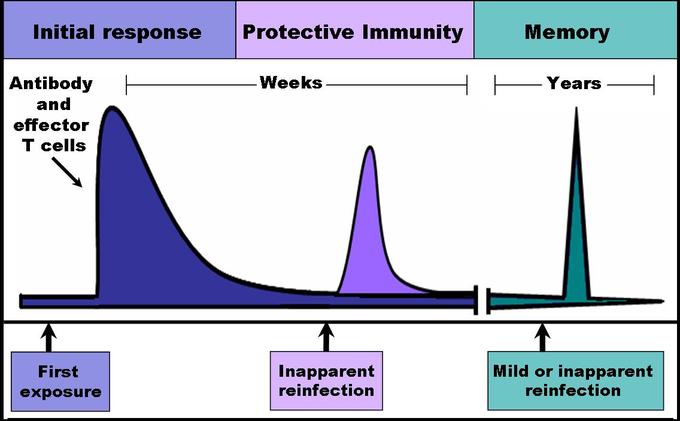
Immune response: When B and T cells begin to replicate, some of the offspring that they produce will end up becoming long-lived memory cells. These memory cells will remember all specific pathogens encountered during the animal’s lifetime and can thus call forth a strong response if the pathogen ever invades the body again.
Passive Memory
Newborn infants are particularly vulnerable to infections since they have no prior exposure to pathogens. Thus, the mother protects the infant through several layers of passive protection. During pregnancy, IgG, a certain isotype of antibody, is transported to the baby from the mother through the placenta, so even babies have high levels of antibodies with similar antigen specificities as the mother. Even breast milk contains antibodies that are transferred to the infant’s gastrointestinal tract and protect against bacterial infections until the baby is capable of making its own antibodies. Since the fetus isn’t making any memory cells or antibodies, this is called passive immunity. Passive immunity is short-lived, ranging from a couple days to a couple months.
As the infant matures, their thymus and bone marrow work to raise a stock of mature lymphocytes that form the foundation for the infant’s personal adaptive immune system. Because the passive memory comes from antibodies instead of B cells themselves, infants do not inherit long-term immunological memory from the mother. Even if the infant receives antibodies specific to certain diseases from its mother, the infant wouldn’t be able to bolster a long-term memory that would direct antigen exposure and presentation.
Active Memory and Immunization
Following an infection, long-term active memory is acquired by activation of B and T cells. Memory cells derive from their parent B and T cells, and undergo clonal selection following infection, which increases antigen-binding affinity. Following reinfection, the secondary immune response typically eliminates the pathogen before symptoms of an infection can occur. During the secondary immune response, memory T cells rapidly proliferate into active helper and cytotoxic T cells specific to that antigen, while memory B cells rapidly produce antibodies to neutralize the pathogen. Long-term active memory consists of rapid response and form permanent immunological memory so long as those memory cells survive.
Vaccinations take advantage of memory lymphocyte development by artificially-generating active immunity, a process called immunization. During a vaccination, the antigen of a pathogen is introduced into the body and stimulates the immune system to develop a specific immunity against that pathogen. It doesn’t cause the disease that the pathogen brings because the vaccine uses an attenuated form of the pathogen that contains the same antigen but doesn’t have the capacity for replication. This deliberate introduction of the pathogen is successful since it exploits the immune system’s natural specificity and inducibility. Vaccination is an extremely effective manipulation of the immune system that helps fight diseases. Over the course of vaccine development, they have saved countless lives, and diseases like rubella and polio are not the widespread causes of disability they once were.
Despite the effectiveness of vaccines, methods do not yet exist to develop vaccines for every pathogen. Many pathogens undergo mutations that change the expression of their antigens, making immunization attempts fruitless for diseases like the common cold or norovirus. Many parasitic pathogens, such as the plasmodium protist that causes malaria, haven’t successfully been vaccinated against because it is challenging to develop a vaccine that is strong enough to stimulate an immune response (sufficient immunogenicity) without causing a live infection.
Major Histocompatibility Complex Antigens (Self-Antigens)
The major histocompatibility complex (MHC) is a cell surface molecule that regulates interactions between white blood cells and other cells.
Learning Objectives
Describe major histocompatibility complex antigens
Key Takeaways
Key Points
- Major histocompatibility complex (MHC) is a cell -surface molecule that mediates interactions between white blood cells and other leukocytes or cells.
- The MHC is divided into class I, class II, and class III subgroups, all encoded by the same gene.
- MHC antigen presentation gains its diversity from the high degree of polymorphism of the MHC protein itself.
- Differing HLA between members of the same species is the main reason why transplanted organs are rejected by the organ recipient.
Key Terms
- human leukocyte antigen (HLA): The name of the major histocompatibility complex (MHC) in humans.
Major histocompatibility complex (MHC) is a cell-surface molecule encoded by a large gene family in all vertebrates. MHC molecules mediate interactions of leukocytes with other leukocytes or body cells. MHC determines compatibility of donors for organ transplant as well as one’s susceptibility to an autoimmune disease via cross-reacting immunization. In humans, MHC is also called human leukocyte antigen (HLA), because MHC can often act as an antigen for human leukocytes.
Types of MHC
MHC is a polymorphic protein attached to the surface of cells. Three different classes of MHC differ in structure and function in the immune system, but are all encoded by the same set of gene sequences.
- MHC I is presented on all cells of the body. It contains an epitope that forms the structural binding site for an antigen. MHC I interacts with natural killer (NK) cells and cytotoxic T cells to signal whether a cell is self or non-self, and whether it contains an antigen specific to that T cell.
- MHC II is presented mainly on macrophages, dendritic cells, and helper T cells, which are all involved in antigen presentation. It has a longer helical region than MHC I, which allows it to bind to CD4 (helper T cells) during antigen presentation.
- MHC III is a secreted enzyme that is neither membrane-bound nor involved in antigen presentation like MHC I and II. It is merely included as an MHC protein because it is encoded by the same set of genes. It is involved in production of complement proteins and inflammatory cytokines.
During antigen processing prior to presentation, protein peptides from pathogens and MHC (I or II) travel through the cytoplasm and then to the cell membrane surface. A complex series of vesicles enables MHC transport from the cell, and the endoplasmic reticulum and Golgi bodies facilitate this transport during antigen processing. After processing, the MHC can present the peptide antigen bound to it to naive T cells.
MHC Class I processing: MHC class I pathway: proteins in the cytosol are degraded by the proteasome, liberating peptides internalized by TAP channel in the endoplasmic reticulum, there associating with MHC-I molecules freshly synthesized. MHC-I/peptide complexes enter Golgi apparatus, are glycosylated, enter secratory vesicles, fuse with the cell membrane, and externalize on the cell membrane interacting with T lymphocytes.
MHC Class I: MHC class I protein molecule.
MHC Class II: MHC class II protein molecule.
HLA and Organ Rejection
Organ transplantation is a complex procedure that can potentially cure many chronic diseases and acute injuries. However, surgically-replaced organs are often rejected by the body’s immune system. MHC is also called human leukocyte antigen (HLA) and varies considerably among different members of the same species. If the T and B cells of the body recognize the HLA of the graft as foreign, they will attack the organ graft. The damage in organ rejection can be acute or chronic, cell-mediated or antibody -mediated, and often involves diffuse damage of the graft that cause necrosis and infarction ( tissue death from lack of oxygen) to the graft tissue by attacking its vascular components.
In nearly all cases, immunosuppressive chemotherapy is a requirement for successful organ transplantation. These drugs can stop acute organ rejection after the procedure, but will not stop chronic organ rejection, in which gradual vascular lesions and endothelial thickening slowly kill the graft. If an organ donor has HLA similar to that of the recipient, the risk of organ rejection is reduced. However this isn’t feasible for heart, liver, or lung transplants because there generally isn’t enough time in these cases to find a matching organ donor.
https://courses.lumenlearning.com/boundless-ap/chapter/humoral-immune-response/
………..left off, learning to say this stuff in regular words still;
Once the adaptive immune system has vanquished the invader, a pool of long-lived memory T and B cells are made. These memory lymphocytes remain dormant until the next time they encounter the same pathogen. This time, though, they produce a much faster and stronger immune reaction. Memory is the key feature of the adaptive immune system, enabling long-term protection…
…This suggests that antibodies to COVID may not last very long. But this does not exclude the existence of memory T and B cells, capable of re-emerging from their dormant states to protect against re-infection. In other words, the antibodies that B cells make during initial exposure disappear in a few weeks, but the memory cells generated as a consequence of this persist for much longer…
Coronavirus: B cells and T cells explained
https://theconversation.com/coronavirus-b-cells-and-t-cells-explained-141888
10-21. Immunological memory is long-lived after infection or vaccination
Most children in the United States are now vaccinated against measles virus; before vaccination was widespread, most were naturally exposed to this virus and suffered from an acute, unpleasant, and potentially dangerous viral illness. Whether through vaccination or infection, children exposed to the virus acquire long-term protection from measles. The same is true of many other acute infectious diseases: this state of protection is a consequence of immunological memory.
The basis of immunological memory has been hard to explore experimentally. Although the phenomenon was first recorded by the ancient Greeks and has been exploited routinely in vaccination programs for over 200 years, it is just now becoming clear that memory reflects a persistent population of specialized memory cells that is independent of the continued persistence of the original antigen that induced them. This mechanism of maintaining memory is consistent with the finding that only individuals who were themselves previously exposed to a given infectious agent are immune, and that memory is not dependent on repeated exposure to infection as a result of contacts with other infected individuals. This was established by observations made on remote island populations, where a virus such as measles can cause an epidemic, infecting all people living on the island at that time, after which the virus disappears for many years. On reintroduction from outside the island, the virus does not affect the original population but causes disease in those people born since the first epidemic. This means that immunological memory need not be maintained by repeated exposure to infectious virus.
Instead, it is most likely that memory is sustained by long-lived antigen-specific lymphocytes that were induced by the original exposure and that persist until a second encounter with the pathogen. It was thought that retained antigen, bound in immune complexes on follicular dendritic cells, might be crucial in maintaining these cells, but recent experiments suggest otherwise. While most of the memory cells are in a resting state, careful studies have shown that a small percentage are dividing at any one time. What stimulates this infrequent cell division is unclear. However, cytokines such as those produced either constitutively or during the course of antigen-specific immune responses directed at noncross-reactive antigens could be responsible. One such cytokine, IL-15, has been implicated in maintaining CD8 memory T cells. Regardless of cell division, the number of memory cells for a given antigen is highly regulated, remaining practically constant during the memory phase.
Immunological memory can be measured experimentally in various ways. Adoptive transfer assays (seeAppendix I, Section A-42) of lymphocytes from animals immunized with simple, nonliving antigens have been favored for such studies, as the antigen cannot proliferate. When an animal is first immunized with a protein antigen, helper T-cell memory against that antigen appears abruptly and is at its maximal level after 5 days or so. Antigen-specific memory B cells appear some days later, because B-cell activation cannot begin until armed helper T cells are available, and B cells must then enter a phase of proliferation and selection in lymphoid tissue. By one month after immunization, memory B cells are present at their maximal levels. These levels are then maintained with little alteration for the lifetime of the animal. In these experiments, the existence of memory cells is measured purely in terms of the transfer of specific responsiveness from an immunized, or ‘primed,’ animal to an irradiated, immunoincompetent and nonimmunized recipient. In the following sections, we will look in more detail at the changes that occur in lymphocytes after antigen priming, and discuss the mechanisms that might account for these changes.
Immunological memory - Immunobiology - NCBI
https://www.ncbi.nlm.nih.gov/books/NBK27158/
Memory T cells survive throughout the lifetime of an individual and are protective upon recall. It is not clear how memory T cells can live so long. Here, we demonstrate that at the resolution of a viral infection, low levels of antigen are captured by B cells and presented to specific CD4+ memory T cells to render a state of unresponsiveness. We demonstrate in two systems that this process occurs naturally during the fall of antigen and is associated with a global gene expression program initiated with the clearance of antigen. Our study suggests that in the absence of antigen, a state of dormancy associated with low energy utilization and proliferation can help memory CD4+ T cells to survive nearly throughout the lifetime of mice. The dormant CD4+ memory T cells become activated by stimulatory signals generated by a subsequent infection. We propose that quiescence might be a mechanism necessary to regulate long-term survival of CD4 memory T cells and to prevent cross-reactivity to self, hence autoimmunity.
Resolution of infection promotes a state of dormancy and long survival of CD4 memory T cells
https://www.ncbi.nlm.nih.gov/pmc/articles/PMC3131418/
Figure 1. The generation of memory B cells and plasma cells in a T-dependent response (based on mouse studies). (a) Antigen-activation brings B- and T cells in contact at the T-B border in secondary lymphoid organs. (b) After initial proliferation in the outer follicle, the B cells make their first fate choice: (1) differentiation into extrafollicular (mostly short-lived) plasma cells (higher affinity), (2) differentiation into very early memory B cells (lower affinity), or (3) up-regulation of Bcl-6 and formation of a germinal center (GC). (c,d) In the GC, a similar selection process takes place in the light zone (LZ). Here, high-affinity LZ GC B cells receive strong T-cell help and consequently down-regulate Bach2 and Bcl-6 while turning on the plasma cell transcriptional program (Blimp-1, IRF-4, XBP-1; including up-regulation of CXCR4) (c). The plasma cell precursors will then either enter the circulation as short-lived antibody-secreting cells, or they will upregulate CXCR3, CXCR4, and CXCR5 to allow migration to the bone marrow plasma cell niche (d). Here survival factors produced by stromal cells and other adjacent cells (including eosinophils and macrophages) promote their differentiation into long-lived plasma cells, which continue to secrete antibodies for the duration of the lifetime of the host. (e,f) Due to the weaker T-cell help received by low-affinity LZ GC B cells, these will not be instructed to turn on either the plasma cell or the GC B cell transcriptional program. Instead, up-regulation of Bach2, CCR6, EBI2, Ephrin-B1, and IL-9R, together with down-regulation of Bcl-6 and S1PR2, promote differentiation to memory B cells (e). To maximize the likelihood of secondary antigen encounter memory B cells will then position themselves strategically in secondary lymphoid organs, become tissue-resident at the site of infection, or patrol as recirculating cells (f).
Frontiers | Remembrance of Things Past: Long-Term B Cell Memory After Infection and Vaccination | Immunology
https://www.frontiersin.org/articles/10.3389/fimmu.2019.01787/full
Left off on long lived b cells or plasma cells
https://en.wikipedia.org/wiki/Plasma_cell
……………
Germinal centers or germinal centres (GCs) are transiently formed structures within B cell zone (follicles) in secondary lymphoid organs – lymph nodes, ileal Peyer's patches, and the spleen – where mature B cells are activated, proliferate, differentiate, and mutate their antibody genes (through somatic hypermutation aimed at achieving higher affinity) during a normal immune response; most of the germinal center B cells (BGC) are removed by tingible body macrophages. The B cells develop dynamically after the activation of follicular B cells by T-dependent antigen.
https://en.wikipedia.org/wiki/Germinal_center
https://en.wikipedia.org/wiki/Immunological_memory
https://en.wikipedia.org/wiki/Memory_B_cell
The mantle zone (or just mantle) of a lymphatic nodule (or lymphatic follicle) is an outer ring of small lymphocytes surrounding a germinal center. It is also known as the "corona". It contains transient lymphocytes. It is the location of the lymphoma in mantle cell lymphoma.
https://en.wikipedia.org/wiki/Mantle_zone
A tingible body macrophage is a type of macrophage predominantly found in germinal centers, containing many phagocytized, apoptotic cells in various states of degradation, referred to as tingible bodies (tingible meaning stainable). Tingible body macrophages contain condensed chromatin fragments.
It is thought that they may play a role in downregulating the germinal center reaction by the release of prostaglandins and hence a reduced B-cell induction of IL-2.
Macrophages that contain debris from ingested lymphocytes are characteristic of a reactive follicular center in benign reactive lymphadenitis. Other accompanying signs of a benign follicular hyperplasia are well developed germinal centers with dark and light zones, in addition to numerous mitotic figures.
https://en.wikipedia.org/wiki/Tingible_body_macrophage
……………………..
V(D)J recombination is the mechanism of somatic recombination that occurs only in developing lymphocytes during the early stages of T and B cell maturation. It results in the highly diverse repertoire of antibodies/immunoglobulins and T cell receptors (TCRs) found in B cells and T cells, respectively. The process is a defining feature of the adaptive immune system.
V(D)J recombination in mammals occurs in the primary lymphoid organs (bone marrow for B cells and thymus for T cells) and in a nearly random fashion rearranges variable (V), joining (J), and in some cases, diversity (D) gene segments. The process ultimately results in novel amino acid sequences in the antigen-binding regions of immunoglobulins and TCRs that allow for the recognition of antigens from nearly all pathogens including bacteria, viruses, parasites, and worms as well as "altered self cells" as seen in cancer. The recognition can also be allergic in nature (e.g. to pollen or other allergens) or may match host tissues and lead to autoimmunity.
In 1987, Susumu Tonegawa was awarded the Nobel Prize in Physiology or Medicine "for his discovery of the genetic principle for generation of antibody diversity".
V(D)J recombination - Wikipedia
https://en.wikipedia.org/wiki/V(D)J_recombination
B cells undergo two types of selection while developing in the bone marrow to ensure proper development, both involving B cell receptors (BCR) on the surface of the cell.
………….
Positive selection occurs through antigen-independent signaling involving both the pre-BCR and the BCR. If these receptors do not bind to their ligand, B cells do not receive the proper signals and cease to develop.
Negative selection occurs through the binding of self-antigen with the BCR; If the BCR can bind strongly to self-antigen, then the B cell undergoes one of four fates: clonal deletion, receptor editing, anergy, or ignorance (B cell ignores signal and continues development).
………….
This negative selection process leads to a state of central tolerance, in which the mature B cells do not bind self antigens present in the bone marrow.[6]
To complete development, immature B cells migrate from the bone marrow into the spleen as transitional B cells, passing through two transitional stages: T1 and T2. Throughout their migration to the spleen and after spleen entry, they are considered T1 B cells. Within the spleen, T1 B cells transition to T2 B cells. T2 B cells differentiate into either follicular (FO) B cells or marginal zone (MZ) B cells depending on signals received through the BCR and other receptors.[ Once differentiated, they are now considered mature B cells, or naive B cells.
V(D)J recombination - Wikipedia
https://en.wikipedia.org/wiki/V(D)J_recombination
In the developing B cell, the first recombination event to occur is between one D and one J gene segment of the heavy chain locus. Any DNA between these two gene segments is deleted. This D-J recombination is followed by the joining of one V gene segment, from a region upstream of the newly formed DJ complex, forming a rearranged VDJ gene segment. All other gene segments between V and D segments are now deleted from the cell's genome. Primary transcript (unspliced RNA) is generated containing the VDJ region of the heavy chain and both the constant mu and delta chains (Cμ and Cδ). (i.e. the primary transcript contains the segments: V-D-J-Cμ-Cδ). The primary RNA is processed to add a polyadenylated (poly-A) tail after the Cμ chain and to remove sequence between the VDJ segment and this constant gene segment. Translation of this mRNA leads to the production of the IgM heavy chain protein.
https://en.wikipedia.org/wiki/V(D)J_recombination#Heavy_chain